Solar Gains Control Systems
Commercial Systems
LCW Switchable Windows
By Philippe Lemarchand and Noel O’Neill, Technological University Dublin, Ireland
Product Description
Brief Concept Description
The Liquid Crystal Window (LCW) technology developed by Merck, known as Licrivision® (Merck, 2019a) and EyriseTM (Merck, 2019b) for glass in architectural application, uses nematic liquid crystals combined with light absorptive material to form switchable windows. Such windows electronically vary daylight transmission without impairing the view through the window. The change of transparency to any given transmission between its high transmission (clear) state and its low transmission (opaque) state occurs within seconds. With regard to solar radiation control, shading and glare are dynamically controlled. The smart operation of LCWs lowers the energy consumption associated to indoor lighting, heating and cooling and the associated carbon dioxide emission and operational costs (especially during the cooling season) and improve the occupants’ health and comfort. Moreover, with a choice of the window colour appearance, a wide range of architectural aesthetic can be achieved.
Architectural and Technological Integration into the Envelope
LCWs can enhance the design freedom of architects and building designers and allow the development of more creative and potentially larger glazed building facades, since they replace other shading devices installed for glare and sun protection. LCWs are façade integrated and will resemble any normal glazed façade, although a range of window colours is available to widen the range of architectural aesthetics that can be achieved. LCWs have no moving or mechanical parts and thus have very low maintenance needs and allow a long-term operation.
The need for LCWs for sun protection will depend on the orientation of the façade and the intensity of the solar radiation, but in general they can be installed on any façade orientation with no specific restriction. Depending on the desired solar shading level and on the required illuminance in the interior spaces, LCWs will range in light intensity from translucent to fully transparent.
One particular advantage is the fast responsiveness of the system, and this is especially beneficial in maritime climates or in high- to mid- latitude European countries such as the Netherlands, where external temperatures can quickly change over the course of the day. LCWs can rapidly and directly respond to the quick changes in the external climate, giving a more stable indoor environment while reducing peak loads and therefore enabling the use of slower responsive HVAC systems that use the buildings fabric to store / release heat (e.g. concrete core activation, under floor heating etc.).
Integration into the Building: System and Comfort
LCWs can effectively control and lower the amount of solar energy that penetrates the glass façade. A correct management of daylighting and indoor glare levels can improve the comfort and reduce the electricity consumption of artificial lighting. Moreover, LCWs electronically vary daylight transmission without impairing the view through the window and research in the field of human comfort has shown that a view on the external environment is healthy, improves the productivity and gives occupants a feeling of comfort and overall wellbeing.
Furthermore, an optimized control of solar gains means significant cost savings over the life of a building due to both a lower capital investment cost for the HVAC system (i.e. reduced size of the energy system) and savings in its annual operational costs. It is understood that LCWs can effectively manage solar gains but an auxiliary heating / cooling system will be needed in most buildings. In this case, the controls of the building system need to be configured so that the LCWs are used to reduce the amount of solar energy entering the building while balancing the need for indoor artificial lighting. Thermal comfort is then adjusted with the use of an auxiliary air conditioning system to meet the buildings heating / cooling demand.
To achieve a complete integration with the building’s heating, ventilation and electrical controls system, LCWs require a good coordination in the building design team since the early phases of the design process and an ongoing technical support for the building contractors, with particular attention required by glazed panel, framing equipment and electrical controls. Recent developments have shown that wired glass is becoming more common in framing systems (a well-known wired glass example is Alarm-glass).
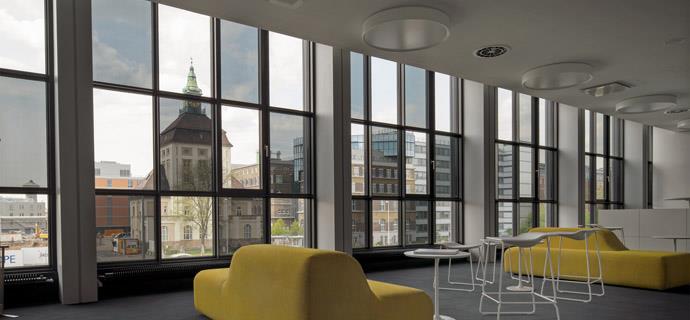
Figure 50. Colour neutral LCWs in low and high transmission states viewed from the indoor environment.
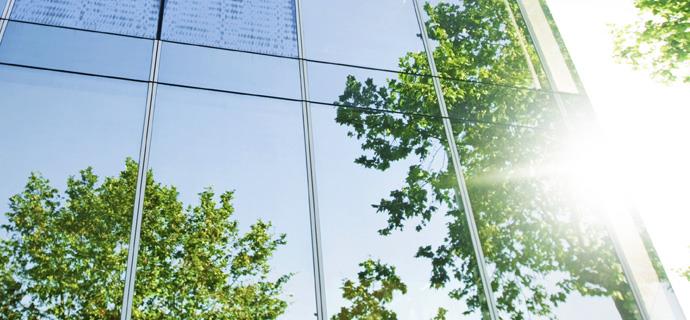
Figure 51. LCWs viewed from the external environment.
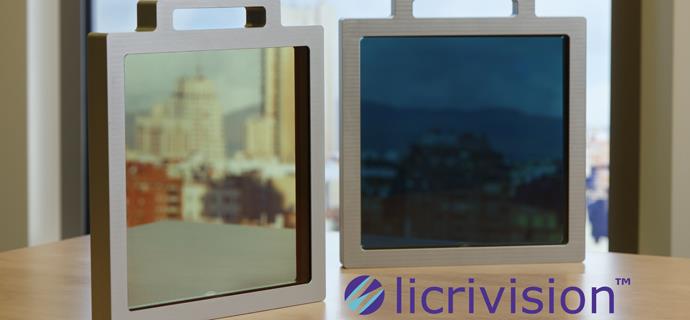
Figure 52. Blue-tint LCWs samples in high and low transmission states.
SWOT Analysis
Strengths
- It allows a glazed façade appearance, suitable for cityscape architectural applications with only a little impact on the façade thickness or weight
- The architectural integration is easy, as it is similar to existing glazed framing systems
- Solar heat gains can be effectively controlled reducing the buildings’ energy and operational cost and capital investment associated to heating, cooling and artificial lighting systems
- It can be combined with any glass coating to improve the building’s energy performance
- Maintenance costs are low, as there are no moving or mechanical parts
- The system is simple and easy to understand
- The operation is quiet and can be used in city environments not suited for openable windows
Weaknesses
- The technology is recent and no research is available on the impact of long-term use of LCWs on a building’s façade
- The life span of LCW façade solutions is hard to quantify
- The façade framing system needs to be designed to allow electrical wiring
- The investment cost is high compared to conventional active shading solutions
Opportunity
- There is a strong movement towards net zero energy buildings (NZEB), supported by legal obligations where LCWs could be used to lower buildings’ thermal and electrical energy needs
- Other uses of LCWs are possible, e.g. windows for cars and security to ensure windows are opaque during out of hour’s operation
- LCWs can be integrated with other smart devices, monitoring and controls to form intelligent and adaptive buildings accounting for the occupants’ needs and requirements
- LCWs controlling solar transmission by absorption can be combined with Phase Change Materials for heat storage
- Existing glazed building façades can be upgraded with LCWs
Threats
- The construction / building sector is risk-conscious and slow to adopt new untested technologies, preferring instead existing solutions with a proven record of accomplishment
- The business case is partly based on ‘intangible’ values such as comfort level, occupant view and daylighting levels
- The integration of LCWs’ control with the HVAC controls in the Building Management System can be complex. The successful application requires a multidisciplinary approach where the façade system, electrical controls and the HVAC systems are all designed to operate and respond with one another
Lessons Learned
The properties of chromogenic windows can be compared with the requirements for switchable windows to be applied to solar energy and solar applications (Beatens et al., 2010) summarised in Table 1.
The properties and attributes of the Licrivision® LCW are shown in Table 2 and Table 3 respectively. A continuous control over the orientation of the molecules, ergo the optical absorption of the device, is obtainable by applying a voltage. The window can be varied from 11% to 52% light transmission in the visible light range, thereby satisfying the requirements from Table 1 and provide controllable indoor lighting and glare conditions. The solar energy transmittance of the window can then vary to a G-value from 0.20 to 0.37 (corresponding to an SHGC variation from 0.23 to 0.39) and enable dynamic control over the solar heat gain. The response time to switch on and off a window is typically below a second. According to Merck, the life cycle capability of the LCW is above 106 cycles. This is also very satisfying as it matches the upper limit requirement in Table 1. This device has been cycled between -20ºC and 70ºC making then suitable for indoor and outdoor environments for building applications. The main drawback to LC devices is their continuous and need for power (only 2 W/m2 in the high transmission state) due to their operating voltage (24 VAC) required to maintain the transparent state.
Competitive technologies to the LCW include electrochromic (EC) windows (Gesimat, 2015a,b; EControl, 2018; SageGlass, 2010, 2013) and suspended particle devices (SPD) (Innovative Glass Corp, 2013; SmartGlass, 2011). Both LCW and SPD require constant power to maintain the desired transmission and inherit a low transmission modulation over the solar spectral range (300-2500nm). EC switchable windows maintain the desired transmission without the need of power and typically provide a transmission modulation over the solar range between 4% and 41% (IGU) corresponding to a variation of the solar energy transmittance (SHGC) from ~0.1 to 0.49. Therefore, EC windows are currently advantageous regarding those aspect. All technologies have a specular transmittance. They have an upper operating temperature limit in order of ~60ºC and are therefore most suitable for building facades. However, the advantage of LCW and SPD over EC is its fast switching capability (below 1 second versus 120-180 seconds for similar sizes) making LCW and SPD more appropriate when a fast visual transmission change is required; in particular for 1) controlling indoor lighting in variable outdoor weather conditions and 2) avoiding glare in a shorter response time. The advantage of LCW over SPD is in the choice of colour and colour neutrality, providing a range of architectural choices and indoor lighting conditions.
Further Reading
Baetens, R., Jelle, B. P. & Gustavsen, A. (2010). Properties, requirements and possibilities of smart windows for dynamic daylight and solar energy control in buildings: A state-of-the-art review. Solar Energy Materials and Solar Cells, 94, pp. 87-105.
EControl-Glas (2018). EControl: Solar Control with Dimmable Glass [online] Available from https://www.econtrolglas.de/fileadmin/econtrol-glas/dateien/technische_dokumente/Broschuere_EN_2018.pdf [Accessed 2019].
Gesimat Gmbh (2015)a. Charakteristische Werte für Gesimat EC elektrochrome Isoliergläser (Beispiele). [online] Available from http://www.gesimat.de/data/Daten_GesimatEC-Iso.pdf [Accessed 2016].
Gesimat Gmbh (2015)b. Elektrochrome Verbundgläser. [online] Available from http://www.gesimat.de/data/produktinformation-EC.pdf [Accessed 2016].
Granqvist, C. G. (2015). Thermochromic and electrochromic fenestration: New nanotechnologies for energyefficient buildings. In: CISBAT 2015. Lausanne, Switzerland: EPFL, pp. 29.
Innovative Glass Corp. (2013). SPD SmartGlass. [online]. Available from https://innovativeglasscorp.com/ourproducts/spd-smart-glass/ [Accessed 2019].
Merck (2019)a. Licrivision® Solar [online] Available from https://www.merckgroup.com/en/s/licrivision.html
Merck (2019)b. EyriseTM Dynamic Liquid Crystal Windows [online] Available from https://www.merckgroup.com/content/dam/web/corporate/non-images/business-specifics/performancematerials/Architecture/global/eyrise_s350_brochure_EN.pdf
SageGlass (2010). SageGlass Product. [online] Available from www.sageglass.com/eu/products/sageglass [Accessed 2019].
SageGlass (2013). Special Function Glazing - Section08 88 00. [online] Available from www.sageglass.com/sites/default/files/sageglass_section088800_special_function_glazing_nonproprietaryversion.docx [Accessed 2019].
SmartGlass International Ltd. (2011). Electronically Switchable Glass Handbook. [online] Available from http://smartglassinternational.com/downloads/SmartGlass_Handbook_2012.pdf [Accessed 2019].